|
Chapter 1: Introduction |
Contents:
|
Introduction

 |
By
definition, a system is composed of interrelated parts.
In systems theory, the degree of interrelationship is termed
the "wholeness" of the system. If the operation of
every part of a system is related to every other part,
wholeness is said to be high. And in fact, an outcome
measure taken from any part of such a system will represent
the effectiveness of every part of the system to the
extent that other parts enter into the outcome. Because all
parts are interrelated, all of the outcome measures taken
from this system will be complex measures reflecting the
operation of every other part of the system and will be
substantially intercorrelated.
For logical purposes, it is useful to contrast a system
of high wholeness to a nonsystem in which no parts
are interrelated. Measures of outcome would not
reflect the operation of other parts measured and would not
be Intercorrelated. This is so obvious that it seems
silly. But the converse, stated above, is not so easily
grasped: outcome measures from different parts of a system
are correlated because those outcomes are jointly
determined by common parts of the system.
What we hope is obvious is that the parts of the system
themselves are interrelated but are theoretically
independent in their unique operation. The only way to
demonstrate this independence is to obtain less complex
measures of outcome of that particular part of the
system which are free of the effects of other parts of the
system. As an example, the quality of the library staff
would be one variable contributing to library size, A test
of librarianship skills could be devised and administered to
the library staff It would certainly be expected that the
results of this test would be less correlated with
university quality than would be library size. That is,
the more molecular the measure, the less intimately it would
be expected to he related to global indices of system
functioning. However, more molecular measures would give
more specific information about system functioning,
We believe the same holds true for mental ability.
Certainly the human mind is a well‑integrated system
having a high degree of wholeness. Wholeness is
reflected in complex measures of human ability, which
explains the high correlations between standard tests of
intelligence. Simpler, more molecular measures should be
individually less highly correlated with more complex
measures but should provide more specific information about
the operation of the system.
Management sciences
have learned a great deal about organizations and how they
work. Much of this learning has come from adopting the
perspective that organizations are entities (systems,
defined later), much like people,
plants and animals. There are many benefits to leaders who
adopt this systems view of their organizations.
Systems thinking has its foundation in the field of system dynamics,
started
in 1956 by MIT professor Jay Forrester.
Professor Forrester recognized the need for a better way
of testing new ideas about social systems, in the same
way we can test ideas in engineering. Systems thinking
allows people to make their understanding of social systems
explicit and improve them in the same way that people can
use engineering principles to improve their understanding of
mechanical systems.
Systems thinking are fast becoming a powerful tool for
decision-making and organizational change. All employees
in a company should be equipped with the skills necessary
for systems thinking. It is imperative to have some
awareness of the origin of systems thinking and how it
can be of benefit to various types of organizational
change, such as reengineering, systems integration,
process redesign, Total Quality Management, and teamwork.
In order to apply systems thinking to challenges that occur
in the work place, some of the tools and methodologies used
in systems thinking should be taught. Some of the best known
strategies used to implement systems thinking include
systems modelling, simulations, causal loops, archetypes,
and scenario planning. To meet the complex changes that are
inevitable, systems thinking can no longer be esoteric
knowledge held by few managers, but should be accessed by
all.
The
approach of systems thinking is fundamentally
different from that of traditional forms of analysis.
Traditional analysis focuses on separating the individual
pieces of what is being studied; in fact, the word
“analysis” actually comes from the root meaning “to
break into constituent parts.” Systems thinking,
in contrast, focuses on how the thing being studied
interacts with the other constituents of the system – a
set of elements that interact to produce behavior – of which
it is a part.
The character of systems thinking makes it extremely
effective on the most difficult types of problems to solve:
those involving complex issues, those that depend a great
deal on the past or on the actions of others, and those
stemming from ineffective coordination among those involved.
Examples of areas in which systems thinking has proven its
value include:
§
Complex problems that involve helping many actors see the “big
picture” and not just their part of it.
§
Recurring problems or those that have been made worse by past attempts
to fix them.
§
Issues where an action affects (or is affected by) the environment
surrounding the issue, either the natural environment or the
competitive environment.
System
Thinking stresses the systemic pattern of thinking (Systemic
is the attribute of thinking derived from systems approach) |
1.1 Basic
Definitions
 |
A
system
• Is an object/process that has components bound by a
mission and has a surrounding environment.
• Is an ordered, interdependent assemblage of components in
a field that has boundaries defined by a clear mission.
Systems Thinking
• Is seeing through “the system’s structure generating
changes and creating the problems.”
• Is a global way of thinking taking into considerations all
factors bounded by the mission of the system. |
1.2 What is a
System?
 |
Very simply, a system is a collection of parts (or
subsystems) integrated to accomplish an overall goal (a
system of people is an organization). Systems have input
processes, outputs and outcomes, with ongoing feedback among
these various parts. If one part of the system is removed,
the nature of the system is changed.
Systems range from very simple to very complex. There are
numerous types of simple systems. For example, there are
biological systems (the heart, etc.), mechanical systems
(thermostat, etc.), human/mechanical systems (riding a
bicycle, etc.), ecological systems (predator/prey, etc.),
social systems (groups, supply and demand, friendship, etc.)
and psychological systems (memory, thinking… etc.).
Complex systems, such as social systems, are comprised of
numerous subsystems, as well; These subsystems are arranged
in hierarchies, and integrated to accomplish the goal of the
system. Each subsystem has its own boundaries of sorts, and
includes various inputs, processes, outputs, and outcomes
geared to accomplish and overall goal for the subsystem.
A pile of sand is not a system. If one removes a sand
particle, you have still got a pile of sand. However, a
functioning car is a system. Remove the carburetor and you
have no longer got a working car.
Importance
of Looking at
Organizations as Systems
 |
1.2.1 Importance of
Looking at Organizations as Systems
The
effect of this systems theory in management is that
it helps managers to look at organizations from a
broader perspective. In the past, managers typically
took one part and focused on it. Then they moved all
attention to another part. The problem was that an
organization could, for example, have wonderful
departments that operate well by themselves but do
not integrate well together; consequently, the
organization suffers as a whole.
Now, more managers are recognizing the
various parts of the organization, and, in
particular, the interrelations of the parts, for
example, the coordination of central offices with
other departments, engineering with manufacturing,
supervisors with workers, etc. Managers now focus
more attention on matters of ongoing organization
and feedback. Managers now diagnose problems, not by
examining what appear to be separate pieces of the
organization, but by recognizing larger patterns of
interactions. Managers maintain perspective by
focusing on the outcomes they want from their
organizations. Now, manager's focus on structures
that provoke behaviors that determine events –
rather than reacting to events as was always done in
the past.
|
Systems Theory, Systems Analysis,
and Systems Thinking

|
1.2.2
Systems Theory, Systems Analysis, and Systems
Thinking
One of the
major breakthroughs in understanding the complex
world is systems theory. The application of this
theory is called systems analysis. One of the tools
of systems analysis is systems thinking. Very
basically, systems thinking is a way of helping a
person to view the world, including its
organizations, from a broad perspective that
includes structures, patterns and events, rather
than just the events themselves. This broad view
helps one to identify the real causes of issues and
know where to work to address them. |
|
1.3 Basic
Concept
and
Characteristics
|
1.3 Basic
Concepts and Characteristics
A System |
1.
A system
·
Must have practical boundaries.
·
Can be greater than the sum of its components.
·
Can be closed or open.
· Must have feedback. |
An
Open System
 |
2. Systems
Theory, Systems Analysis, and Systems Thinking
One of the
major breakthroughs in understanding the complex
world is systems theory. The application of this
theory is called systems analysis. One of the tools
of systems analysis is systems thinking. Very
basically, systems thinking is a way of helping a
person to view the world, including its
organizations, from a broad perspective that
includes structures, patterns and events, rather
than just the events themselves. This broad view
helps one to identify the real causes of issues and
know where to work to address them. |
|
Basic
principles

|
Systems theory has identified numerous principles that are
common to systems,
many of which help us to better understand organizations.
Systems
thinking reposes on basic principles:
1. Have boundaries |
Any system must have boundaries
that separate it from its environment. This
principle is essential for studying a system or
improving it. If the system is big, it should be
broken into subsystems with clear, practical
boundaries. |
2. Influence behavior
 |
Structures
influence behavior: when there are problems at
work, mainly because structure elements do not work
together, performance (a result of behavior) fails
to live up with what is planned. People tend to
react in three different ways:
·
Addressing systemic structure
because systems generate behavior (generative
reaction)
·
Addressing patterns of behavior
because behaviors produce events (responsive
reaction).
·
Addressing results or events
when they produce (reactive response: most common
and the easiest way to react). Addressing structures
prevents reproduction of behaviors that result in
problematic events. Therefore, to improve a system,
consider improving the structure that runs this
system. |
3. System volume
 |
A
system can always be more than the sum of its
components. That a system can always include the effect of synergy. If not, then
there is something within not working in harmony
with the other components. There is always a
position where the function of the system is optimum
or effective. This position has to be sought.
Effectiveness is not a static property; it changes
with change of circumstances and external
environment. System effectiveness is apparent when
its outputs exceed the sum of the individual
outputs. This can be accomplished when there is
unity of direction and commonness of objectives of
its members and where teams or individuals in the
organization see where they stand in relation to the
company’s other work, especially in cross-functional
groups.
The
fact that sum of the system can be greater than the
sum of the individual work of its employees, proves
that effective systems have synergy. Such state of
synergy is reached when waste is minimal, and when
all actions add value to the mission of the system. |
4. Closed or opened
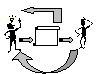 |
A system can be considered closed or open at a
certain period of time.
An open system has some kind of exchange with the
environment. A closed system does not have this
exchange: a system in the universe cannot have any
exchange with the environment unless for a limited
period of time. The car is a closed system, to some
extent, when it is parked and not used. When used,
the car becomes an open system and exchanges certain
product with the environment. |
5. Survival of
open
system
 |
For an open system to survive,
it must ingest enough input from its environment to
offset its output as well as the energy and material
used in its operation. This is referred to as
“steady state.” Steady state conditions are dynamic:
the system must be able to change in order to adapt
to the dynamic situation of the environment of the
system. Before reaching a steady state, system can
be in a re-enforcement state. Re-enforcement can be
positive (if performance is increasing as a result
of positive feedback) or negative (when performance
is decreasing as a result of negative feedback).
Open systems tend to specialize and elaborate their
elements and structure and enlarge their boundaries
with time, with size and maybe with the change of
the environment. |
6. No unique solution
for open system
 |
In open systems, there is no unique solution
to the same problem: there are many ways to produce
the same output or there are many outputs for the
same input. |
7. Feedback
 |
A system must have feedback:
information that the system needs to maintain steady
state and to know that it is not in danger of
destruction. |
8. Cause and effect
 |
In systems thinking, every influence is both
cause and effect: i.e. a cause can also be an effect
of something else when regarded in different way |
|
1.4 Stages of
Systemic Thinking
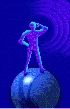
Input output
principle forms


Applying
system approach

Potential
impact on people

Development
Systems
do
not
have to be complicated

Example




How brain
system handled information
Language,
notation and mathematics
Some
necessary properties
Individual's
psychological resources
Concept
of entropy of living organisms
A closed
system

|
1.4 Stages of
Systemic Thinking
The Input-Output technique developed by the American General
Electric Company can be helpful. Although it need not be, its use has been restricted mainly
to technical problems in which the input is energy, light,
heat, electricity, etc. - with a desired output in some way
dependent upon it. Whiting gives, for example, the problem
of devising a fire warning system. The input is fire and the
required output a warning that fire is present, with a
number of constraints in between: the warning must be
foolproof and continuously available; it must be
quick-acting to minimize damage; and it must be discernible
at points remote from the fire. The problem may not be
solved in one step. A warning system requires several
intermediate steps, starting with the fire itself and ending
with some physical warning system. Whiting warns against
trying to short-circuit any intermediate point – this is
more likely to lead to a stereotyped solution, since it
fails to consider the opportunities for branching into the
alternative paths offered by multiple outputs generated at
some stages.
The Input-Output principle forms much of what might be
considered the heart of a ‘Systems’ approach. This removes the limitations of a problem defined in
purely technical terms and extends the definition of input,
output and constraints to include the whole situation – men,
money, materials, machines and methods. It thereby provides
an overall view and allows us to arrive at a more
comprehensive, unified and long-lasting solution than any
piecemeal approach can make possible.
Thus, in applying a system approach, say, to a problem
involving the manufacture of a chemical, we would not be
limited to the technicalities of the process, choice of
materials of construction, design and performance of
mechanical and electrical equipment and methods of
measurement and control. We should, in addition, be involved
with the problems of processing and handling raw materials,
methods of transport, and use and disposal of finished
products; with the recruitment, training and working
conditions of the management and men needed to run the
plant; with the effects of the product and its manufacture
on the local environment – the noise, smell, smoke and
general pollution produced; with the long-term effects of
our presence as an employer and a source of opportunity.
Even then the list is far from complete, but we are
beginning to paint a fuller picture of the total situation
and thereby identify more of the important variables having
claim to consideration alongside those of technology.
Clearly, the more complex a problem and the greater its
potential impact on people, the more appropriate a systems
approach becomes.
But it would surely be wise to consider all but the most
narrowly defined technical problem in a context which
includes the human element, if we wish to avoid unpleasant
reactions and resistance to our solutions when we create
them.
Jenkins suggests that there are four main stages in the systems approach:
analysis, synthesis, implementation and operation.
1.Analysis
|
1. Analysis
What
is the problem and how should it be tackled?
What
is the nature of the primary system in which the
problem is embedded and the wider environment in
which it, in turn, is contained?
What
are the objectives of these respective levels in the
systems hierarchy? Are they stated clearly and are
they consistent with each other?
Has
all relevant information been collected? Have all
constraints been identified (and all false
constraints eliminated)? |
2.
Synthesis
 |
2.
Synthesis What are the expected changes in the systems under
consideration?
How accurate are the forecasts likely to be?
What models can be built of part or the whole of the
situation describing behavior, processes, operating
conditions, etc.?
In what form should these models be – graphical,
tabular or mathematical? Can the models be
manipulated to simulate changes in the system?
What is the optimum for the whole system?
What system is ‘best’, taking all aspects into
consideration with a proper weighting for each? How
reliable is this system and what uncertainties
remain?
What can be done to ensure that the ‘best’ system is realized in
practice? |
3.
Implementation
 |
3. Implementation
Is the final design fully understood, its implementation adequately
planned and its integration into the wider
system properly organized?
Have the design and plan of action been ‘sold’ to users or operators?
Are all changes understood and accepted?
Are there an adequate commissioning plan and a scheme for evaluation
performance? |
4.
Operation
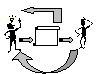 |
4. Operation
Have operation and maintenance procedures been prepared and put into use?
Is there a continuing feedback
of operating experience to designers and are
worthwhile improvements introduced?
Is ultimate obsolescence and replacement catered for?
Techniques of use in such a comprehensive approach include just about
every thing in Management Theory, including Critical
Examination to get the problem right, Critical Path
Scheduling to plan and time the project, Management
by Objectives to define the aims of the whole
venture and to get people committed, Modeling and
Simulation, Risk Analysis, Reliability Studies and
Control Systems to aid design.
Have operation and maintenance procedures been prepared and put into use?
Is there a continuing feedback
of operating experience to designers and are
worthwhile improvements introduced?
Is ultimate obsolescence and replacement catered for?
techniques of use in such a comprehensive approach include just about
every thing in Management Theory, including Critical
Examination to get the problem right, Critical Path
Scheduling to plan and time the project, Management
by Objectives to define the aims of the whole
venture and to get people committed, Modeling and
Simulation, Risk Analysis, Reliability Studies and
Control Systems to aid design. |
A useful development of the Systems approach is given by
Nadler.
He suggests that if we can disengage our thoughts from the
present situation when defining a complex problem and think
instead of an ideal solution, that is, one which is not
restricted by money, method or resources, then by keeping
this ideal solution in mind, we will come nearer to it in
practice than by trying to inch forward with the present as
our reference point. Nadler describes three stages in
the achievement of a workable solution: the Ultimate Ideal
System, the Technologically Workable Ideal System and the
Technologically Workable Ideal System Target. An Ultimate
Idea System represents the best system likely to be
achieved through the development of existing knowledge. But
it is achievable, even though at a later date, and can be
made a target for improvement in the future, giving a fixed
aim point rather than a projection forward from the present
situation. A Technologically Workable Ideal System is
one based on technology which already exists, but which does
not take into account real-life restrictions such as money,
available skill, etc. By designing several systems to this
criterion and selecting one as a guide, a recommended
system, the Technologically Workable Ideal System Target,
as a guide, can finally be described which does take into
account all real-life restrictions.
Systems do not have to be complicated or unintelligible,
or even dressed in jargon. A system is just an
arrangement of circumstances that makes things happen in a
certain way. The circumstances may be metal grids,
electronic components, warm bodies, rules and regulations or
anything else. In each case, what actually happens is
determined by the nature of the system. One can take the
function of the system for granted and become interested in
how it is carried out.
If
young children are asked to invent a potato‑peeling machine
they draw
a‑winding tube through which a string of potatoes is seen
traveling towards a simple box with the explanatory note,
'In here the potatoes are peeled.' Another tube carries the
peeled potatoes away. There is nothing mysterious about the
box; it just performs the potato‑peeling function. One takes
it for granted that is the function of the box and that
somehow the function gets carried out. In some of the
inventions the potatoes are then carried to a metal grid
through which they are forced in order to make chips. The
making of the chips is not taken for granted but explained,
because it is explicable.
If you put water instead of oil into a frying pan you would
not expect to be able to fry chips.
If you were to use fat or oil you would get some ordinary
chips. If you add a little water to the oil before you put
the pan on the fire, then the temperature of the oil will
rise more slowly and the chips will be soft on the inside
and crisp on the outside ‑ much more so than if only the oil
had been used. The nature of the system determines what
happens.
The brain is a system in which things happen according to
the nature of the system.
What happens in the brain is information. And the way how it
happens is thinking.
Since thinking in this broad sense
determines what people do on any level from the most
personal to the most international, it could be worth
looking at some aspects of the brain system.
The first useful thing
that can come out of knowledge of a system is the avoiding
of those errors that arise through thinking the system to be
something that it is not.
The second useful thing
is awareness of the limitations of the system. No matter how
good they may be at performing their best functions, most
systems are rather poor when it comes to performing the
opposite functions. One would no more go racing in a
shopping car than shopping in a racing car. Where one can,
one chooses the system to fit the purpose. More often there
is no choice, and this means that a single system will
perform certain functions well, but others not so well. For
instance, the brain system is well suited to developing
ideas but not
always
so good at generating them. Knowing about the limitations of
a system does not by itself alter them. But by being aware
of the nature of the system one can make deliberate
adjustments.
The third way
in which one could use knowledge of a system would be to
make use of the characteristics of the system to improve its
performance or to achieve some end.
Some understanding of how the brain system handled
information could be very useful.
It might then be possible to recognize some of the errors
and faults inherent in this type of system, to show, for
example, that there was a tendency to arbitrary and
self-enhancing divisions which were extremely useful in most
cases but could also be the source of a lot of trouble.
Apart from becoming aware of the errors of the system, it
might also be possible to make more effective use of it
through understanding its nature in order to make the
learning process easier and more economic. It might be
possible to do something about communication.
Language, notation and mathematics are useful artificial
aids
to thinking. There may be other artificial aids which could
be invented if one had sufficient understanding of the brain
system. With new notation it might prove possible to
generate ideas as easily as we now develop them once they
have been generated. For instance, it might be possible to
invent a new word which would be functional in nature like
'and', 'if', 'but' or 'not. The function of this new word
would be to compensate for the inherent limitations of the
information ‑ processing system in the brain and open up new
ways of talking and thinking. The word would ultimately have
to justify its usefulness in practice, but its invention may
not have been possible without an understanding of the
nature of the system.
There are a few
necessary
properties of systems that need to be stated before
proceeding.
The most basic of these is that systems exhibit some degree
of stability, or constancy. If they do not, it would
not be possible to identify them as the same system over
time. A system may be closed, which means that it is a
self‑contained, self‑regulating entity that is insulated
from, and does not interact with, other systems. Or, it may
be open, or interactive. For open systems to be stable they
must exhibit equilibrium through negative, or compensating,
feedback, because if they do not, their form would change
and the necessary property of stability over time would be
lost. The "hunting" of servo‑mechanisms and the homeostasis
of vegetative biological functions in animals are examples
of open systems maintaining equilibrium through negative
feedback.
The individual's
psychological resources
for coping with the social world‑what we call in everyday
terms the person‑can be construed as a system in this sense.
Analogies with systems in the social sciences usually
concentrate on the behavior of thermodynamic systems. The
second law of thermodynamics states that all closed systems
are subject to increasing entropy. The entropy of a
system is the measure of unavailable energy; energy that
still exists but which is lost for the purpose of doing
work. In thermodynamics, of course, the energy referred to
is heat and the law can be roughly understood as the idea
that all (hot and cold) material within a thermally
insulated area will eventually come to have the same
temperature. The more general version of this principle is
that all closed systems are subject to loss of
differentiation. A correspondence has been established
between the entropy of a system and the loss of information
in that system in the sense of information theory. So, this
principle can also be taken to mean that the information in
a closed system diminishes over time.
Schrödinger, applying the
concept of entropy to living organisms,
writes: "Thus a living organism continually increases its
entropy‑or, as you might say, produces positive entropy‑and
thus tends to approach the dangerous state of maximum
entropy, which is death. It can only keep aloof from it,
i.e., alive, by continually drawing from its environment
negative entropy".
A closed system,
in thermodynamics or biology or whatever, like the concept
of infinity in mathematics, is an ideal or pure state,
unreal when applied to the physical world. But although
closed systems are probably never perfectly realized in
practice, the pure concept serves as a useful anchor for
theories in the study of material things. The same is true
of the concept of system applied to the social world.
Consider, for instance the "ideal" but incredible notion of
a person as a closed system, completely insulated from other
systems, from the rest of the community. Such insulation
would take the form of never talking with anyone and never
doing anything (doing, that is, in the sense of acting and
choosing as non‑automatic, non‑habitual justified
performance). Borrowing from the thermodynamics analogy, one
of the properties of such a closed person‑system would be
its increasing entropy; the gradual decline in the harness
able energy, or differentiation, or information, within it. |
1.5 Understanding the
Nature of the System in Organizations

Good judge
Good grasp
Progress
measurement
Variables to understand the
nature of the system
1. Casual
2. Intervening
3. End-results
Interrelation of variables
Understanding
the nature of the system
Effective managers needs

Important decisions
Other casual relations |
Effective leader‑managers have a common affinity
for understanding the nature of the larger system within
which they work. Whenever they take on new job assignments,
they make a special effort to understand the inner workings
of the larger system of which their work unit is a part.
Realizing that the needed information can not be uncovered
simply from printed documents; they are relentless in their
probing. They observe, inquire, and integrate until they are
satisfied that they have a valid conceptual model of the
system.
John Dewey,
the philosopher and educator, was astute in his portrayal
of the "good judge." This is a person "who has a sense
of the relative indicative or signifying values of the
various features of the perplexing situation; knows what to
let go of as of no account; what to eliminate as irrelevant;
what to retain as conducive to the outcome; what to
emphasize as a clue to the difficulty." In essence, this is
a person who has a profound understanding of the larger
system within which he or she works.
In The Human Organization, Rensis Likert stresses that
the manager should have a good grasp of two aspects of the
system: the nature of the system and the state of the
system. In this regard, he likens the manager's job to
that of the physician:
|
A physician needs
two different kinds of information to make a
correct diagnosis. First, he must know
a great deal about the nature of human beings.
This knowledge is based on extensive research which
relates symptoms to causes and measurements of body
conditions to the health of the organism, thereby
revealing the character of the human body's normal
and abnormal functioning. This knowledge gives the
doctor insights into how the system ought to
function, so that he can know what he needs to
measure and how he needs to interpret the
measurements. The second kind of
information needed by the doctor to discover the
patient's state of health at any particular time is
that revealed by the appropriate measurements and
tests made on that patient at that time. |
It is generally understood that measurement of progress
is dependent on accurately assessing the state of the system
at any point in time. It also must be understood that
accurately assessing the state of the system is dependent on
understanding the nature of the system
To understand the nature of the system,
Likert stresses that the manager must grasp the
relations between and among three types of variables:
1. Causal variables:
independent variables that determine the course of
developments within an organization and
the results
achieved
by the organization.
2. Intervening variables: mediating
variables that reflect the internal state and health
of the organization.
3. End‑result variables: the dependent
variables that reflect the achievements of the
organization. |
As an illustration of how these three classes of variables
interrelate,
we can consider the example of the effect of leadership
style on productivity. In many situations, it would be
assumed that a participative leadership style would be more
effective than an autocratic style. This premise can be
tested by correlating leadership style (causal variable)
with employee motivation (intervening variable), and then
correlating employee motivation with productivity
(end‑result variable). In this way it could be demonstrated
that leadership style has an effect on productivity, but via
employee motivation.
Given this framework for "understanding the nature of the
system", we will illustrate the notion by considering
the dollar flow in a for‑profit engineering firm. You may
not have any particular interest in an engineering firm, but
the principles elucidated here would apply to any type of
organization.
The dollar flow of the illustrative firm is shown in Figure
1.1.
We will consider the business volume to be the causal
variable, the net income to be the end‑result variable, and
everything else to be intervening variables.
The business volume is broken down into these categories:
labour, use of equipment and service centres, all other
project costs, overhead (engineering department overhead,
general overhead, and cost of capital), and fee. The
general overhead is apportioned as direct expenses of
engineering operations (funds allocated to the engineering
departments) and indirect expenses (funds used to operate
the company as a whole).
Effective managers understand the causal relations in this
financial system. For example,
they realize that increasing labour
(time on projects) by one percent can have at least a
10‑percent impact on net income. They realize that a
two‑percent overrun in project losses can cause a 20‑percent
decrease in net income. Further, they realize that a fee
increase of three percent can have a 30‑percent impact on
net income. These multiplier effects are indeed noteworthy,
and they are ever‑present in the mind of the effective
manager.
Figure 1.1: Dollar
flow in an engineering firm
Managers
who understand this financial system also realize that it
presents them with a number of important decisions. For
example, will the return‑on‑investment with the marketing
and proposals funds be better in the industrial arena or in
the government arena? With the funds allocated for internal
research and development, is it better to invest in a small
number of really good ideas or in a large number of possibly
promising ideas? With the funds for associations and
publications, which particular associations and publications
should be pursued? These are important questions, and the
answers generated will determine the success or failure of
the manager.
There are numerous other examples of important causal
relations in the system, but these will illustrate the
point that for you to be able to measure your unit's
progress, it is essential that you understand the inner
workings of the larger system.
|
|
Home
Next Chapter
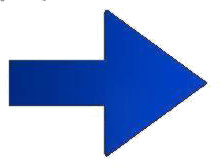
|
|
|
|